Neutron reflectometer-ROG
The ROG neutron reflectometer measures the way neutrons are reflected by flat surfaces and interfaces with the aim to provide information about the thickness, composition and roughness of thin films and other layered structures. It can be used to study (stacks of) layers with thicknesses of 5 - 150 nm while providing a resolution up to 0.2 nm. Neutron reflectometry can be used to non-destructively study both liquid and solid samples in a variety of different experimental conditions.
In a neutron reflectometer, a flat sample is illuminated by a collimated neutron beam under a small angle (< 5 degrees) and the fraction of neutrons reflected relative to the total number of neutrons incident on the sample is determined (i.e. the so-called ‘Reflectivity’).
Similar to the reflections of light and X-rays from a surface, the reflectivity of neutrons depends on the wavelength λ and incident angle θ of the neutron. As illustrated in Fig. 1, for incident angles smaller than the critical angle, all neutrons are reflected. For larger angles, the reflectivity of a material decreases rapidly with increasing angle of incidence. Instead of expressing the reflectivity as a function of the incident angle and/or wavelength, it is typically expressed as a function of the momentum transfer Q of the neutron when reflected by the sample: The momentum transfer incorporates the effect of the incident angle and wavelength in a singly quantity and is related to the angle of incidence and wavelength through.
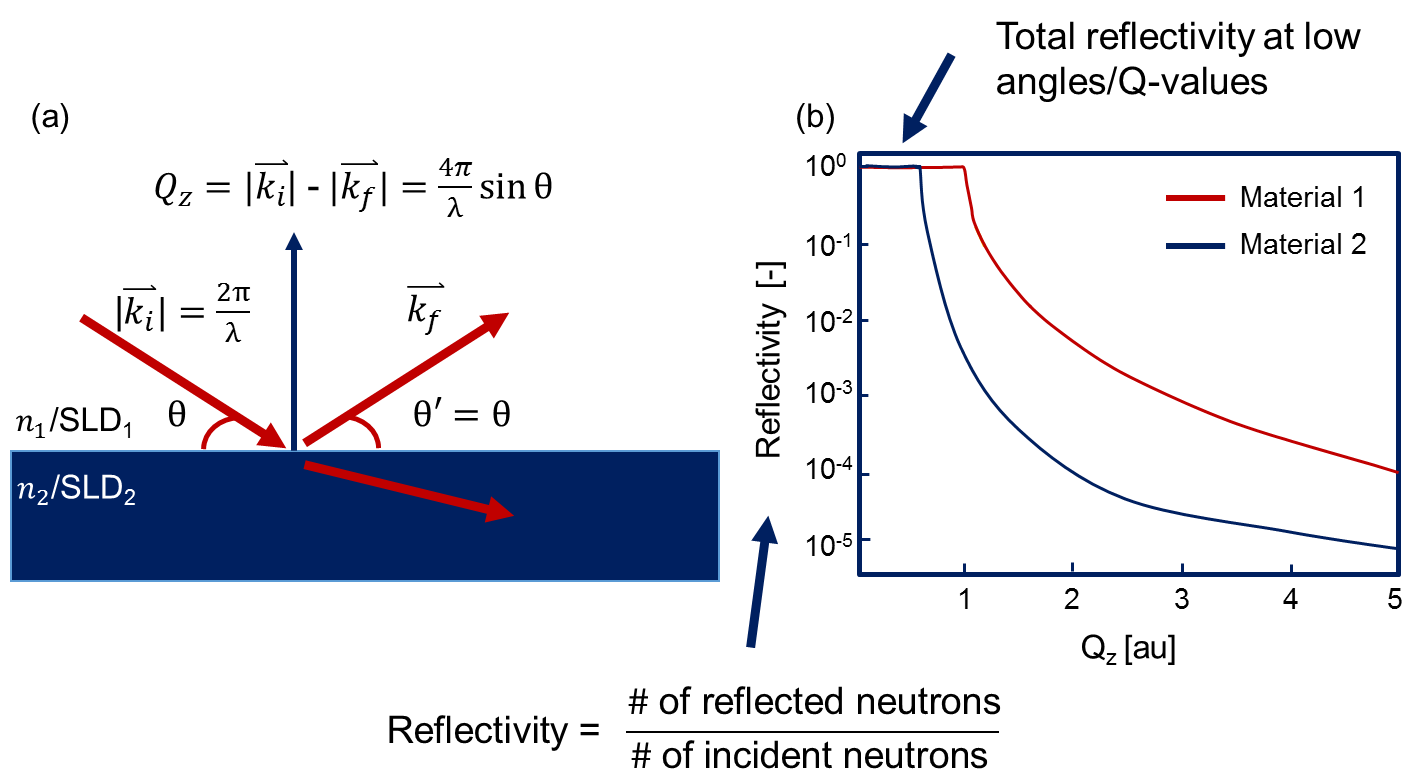
Analogous to the reflection of optical light from surfaces, the critical angle and the subsequent decay of reflectivity with increasing angle of incidence depend on the index of refraction of the material studied. However, for neutrons it is often more convenient to use a different quantity named the Scattering Length Density (SLD). The SLD is the product of the scattering length of a material (an isotope-dependent quantity that is well known) and the number of atoms per volume unit. In contrast to X-rays, the scattering length of neutrons does not monotonously increase with the atomic mass of an element, making neutrons especially powerful for detecting light elements as H and Li and allowing for isotopic substitution (e.g. hydrogen by deuterium).
When a layer of a material is deposited on top of a substrate composed of a material with a different scattering length density, interference occurs between the neutrons reflected from the surface of the sample and neutrons reflected from the interface of the two materials. As illustrated by Figure 2, this interference results in so-called fringes in the reflectivity pattern, whereby the width of the fringes is related to the thickness of the layer and the amplitude to the difference in scattering length density of the two materials.
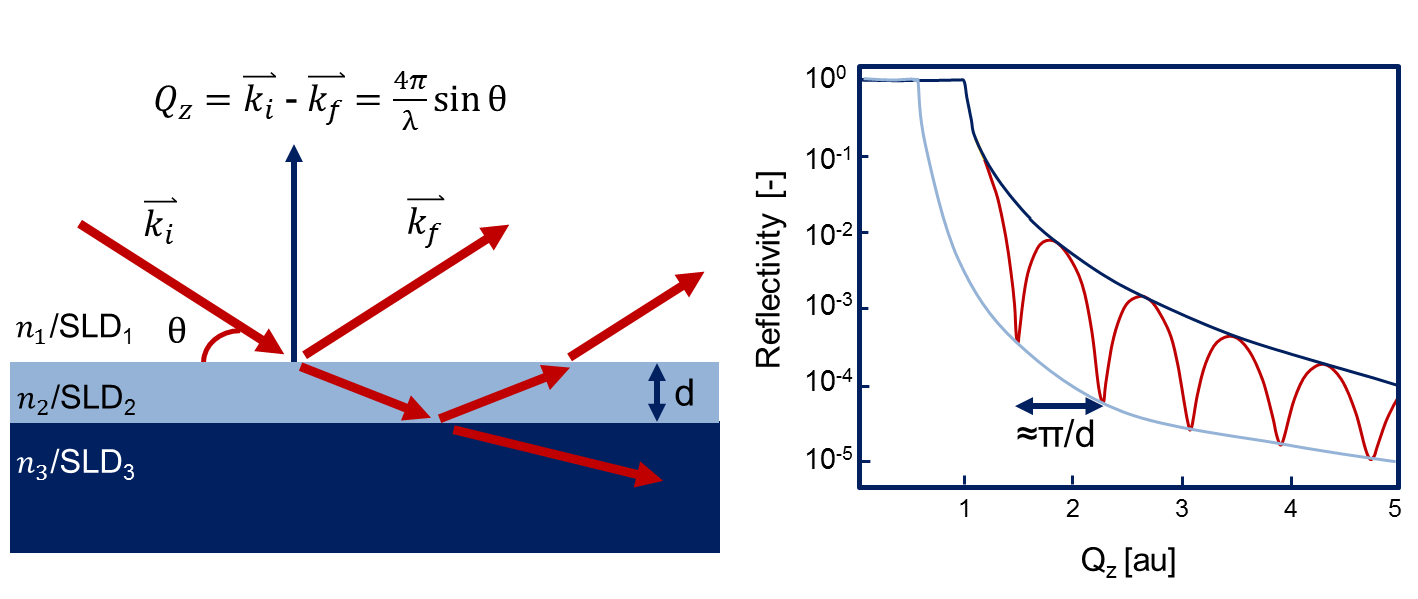
In a typical reflectometry experiment, the Q-dependence of the reflectivity of a sample (can be composed of multiple layers) is determined. Subsequently, the measured reflectivity is fitted to a model system, from which estimates about the layer thicknesses, scattering length density (and thus composition of the material!) and roughness can be obtained.
Applications
- The study of surfaces and interfaces of thin films, multilayers and coatings: e.g. in-situ determination of hydrogen concentration in metal hydrides, in-operando study of battery materials, composition of thin films of polymers.
- Solid-liquid interfaces and membranes: e.g. protein diffusion in membranes, membranes for fuel cells, surfactants, polymers, proteins and enzymes at interfaces, drug delivery systems, study of the interface between a battery electrode and a liquid electrolyte.
- Liquid-air and liquid-liquid interfaces: e.g. proteins, polymers and enzymes in solution,
Example: Thin film metal hydrides as optical hydrogen sensor
- The detection of hydrogen is crucial in many industrial processes and for its adaptation as an energy carrier. Thin film metal hydrides can be used to optically probe the hydrogen pressure without the need for electric currents near the sensing area. These sensors utilize the fact that the optical properties change when the hydrogenation of a thin film metal hydride changes in response to a different partial hydrogen pressure in the environment of the sensor. In order to fundamentally understand the structural response of these thin films to hydrogen, facilitating the rational design of new hydrogen sensors, neutron reflectometry was used to study the temperature and hydrogen pressure dependence of the hydrogen content and thickness of the sensing layer in a variety of thin film metal hydrides (e.g. Hf, Ta, Pd1-yAuy). Neutron reflectometry provided the hydrogen pressure dependence of the hydrogen content in the metal hydride layer as well as the volumetric expansion upon hydrogen sorption and thus enabled a deeper and more fundamental understanding of these materials.
-
The ROG neutron reflectometer is an unpolarized time-of-flight neutron reflectometer with a horizontal scattering geometry that can be used to study both liquid and solid samples in a variety of different experimental conditions. Further specifications of the reflectometer can be found in the table below and in Rev. Sci. Instrum. 94, 113901 (2023) https://doi.org/10.1063/5.0155888
Schematic of the reflectometer in (a) direct beam configuration, (b) configuration for measuring the reflectivity of solids (frame and incoming flight path horizontal, sample and detector tilted), (c) configuration for measuring the reflectivity of liquids (frame and incoming flight path tilted, sample horizontal and detector tilted). (d) Photograph of the ROG neutron reflectometer. (i) chopper located inside the chopper house to create a pulsed neutron beam, (ii) super mirror 1 and super mirror 2 to deflect the neutron beam from the horizontal for liquid/air measurements, (iii) frame that supports and can tilt to allow a sample to be positioned horizontally for liquid/air measurements, (iv) frame-overlap mirror that ensures that slow neutrons with long-wavelengths from the neutron pulse are filtered such that they are not accidentally detected in the next time-of-flight frame with an erroneously determined wavelength, (v) diaphragm 1 and diaphragm 2 to control the angular resolution and the footprint on the sample, (vi) sample compartment with a sample table of which the height and angle can be controlled, (vii) diaphragm 3 to block the direct beam and minimize the background, (viii) detector table of which the angle can be adjusted and on which a neutron prism can be mounted (ix) diaphragm 4 to minimize the background on the detector, (x) the 3He tube detector, and (xi) Position Sensitive Detector (PSD) and beam stop. -
Neutron reflectometry requires large samples with a surface area of at least 400 mm2 (ideally even larger). Most importantly, the samples have to be homogenous in the in-plane direction and extremely flat. Typically, samples for neutron reflectometry are deposited on specially polished (fused) quartz, SrTiO3, MgF substrates or relatively thick (>3 mm) Si wafers. Please contact the instrument scientist for more details.
-
Currently, we have the following sample environment readily available:
- Gas pressure (0 – 10 bar) and temperature (25 – 400 C) controlled cell for in-situ measurements. Journal of Neutron Research 26 (2024) 1-13 https://content.iospress.com/articles/journal-of-neutron-research/jnr230004
- Langmuir though with anti-vibration table
- Laminar flow cell (Physica B 357 (2005) 208-212)
- Overflowing cylinder (Physica B 283 (2000) 278-281)
- Electrochemical Cell (under development)
Not (yet) available what you wish? Please contact the instrument scientist to discuss about the possibilities.
Please check out the info at the page User Office for Proposal Application, Contact Information and FAQ/QA.
Publications
The completely renewed and upgraded neutron reflectometer at the TU Delft Reactor Institute (2023) L.J. Bannenberg, R. Bresser, P. van der Ende, M. van Exter, W. van Goozen, F. Naastepad, M.A. Thijs, M.N. Verleg, C.F. de Vroege, R. Waaijer, A.A. van Well The Review of scientific instruments 94 (11), 113901
Hydrogen Absorption into Copper-Coated Titanium Measured by In Situ Neutron Reflectometry and Electrochemical Impedance Spectroscopy (2023) A Situm, B Bahadormanesh, LJ Bannenberg, F Ooms, HA Feltham, G Popov, M Behazin, LV Goncharova, JJ Noël Journal of The Electrochemical Society 170 (4), 041503
in-situ 3He neutron spin filters at JCNS, status and updates (2023) E Babcock, Z Salhi, A Feoktystov, LJ Bannenberg, SR Parnell, D Alba Venero, V Hutanu, H Thoma, J Xu, P Pistel, J Damean, A Ioffe, S Mattauch Journal of Physics: Conference Series 2481 (1), 012009
Versatile pressure and temperature controlled cell for neutron reflectometry and small-angle neutron scattering (2023) LJ Bannenberg, M van Exter, MN Verleg, B Boshuizen, SR Parnell, MA Thijs, H Schreuders Journal of Neutron Research, 1-13
Completely elastic deformation of hydrogenated Ta thin films (2023) LJ Bannenberg, L Blom, K Sakaki, K Asano, H Schreuders ACS Materials Letters 5 (4), 962-969
Tuning the Properties of Thin-Film TaRu for Hydrogen-Sensing Applications (2023) LJ Bannenberg, H Schreuders, N van Beugen, C Kinane, S Hall, B Dam ACS Applied Materials & Interfaces 15 (6), 8033-8045
Suppression of the Phase Coexistence of the fcc–fct Transition in Hafnium-Hydride Thin Films (2022) LJ Bannenberg, H Schreuders, H Kim, K Sakaki, S Hayashi, K Ikeda, K. Asano, B. Dam The Journal of Physical Chemistry Letters 12, 10969-10974
Anti-bacterial efficacy via drug-delivery system from layer-by-layer coating for percutaneous dental implant components (2019) ED de Avila, AGB Castro, O Tagit, BP Krom, D Löwik, AA van Well, LJ Bannenberg, CE Vergani, JJJP van den Beucken Applied Surface Science 488, 194-204
Optical hydrogen sensing beyond palladium: Hafnium and tantalum as effective sensing materials (2019) LJ Bannenberg, C Boelsma, H Schreuders, S Francke, NJ Steinke, B. Dam Sensors and Actuators B: Chemical 283, 538-548 Direct
Comparison of PdAu Alloy Thin Films and Nanoparticles upon Hydrogen Exposure (2019) L.J. Bannenberg, F.A.A. Nugroho, H. Schreuders, B. Norder, T.T. Trinh, N.J. Steinke, A.A. van Well, C. Langhammer, B. Dam ACS Applied Materials & Interfaces 11 (17), 15489-15497
Hafnium—an optical hydrogen sensor spanning six orders in pressure (2017) C Boelsma, LJ Bannenberg, MJ Van Setten, NJ Steinke, AA Van Well, B. Dam Nature communications 8, 15718
Impact of nanostructuring on the phase behavior of insertion materials: The hydrogenation kinetics of a magnesium thin film (2016) L.J. Bannenberg, H. Schreuders, L. van Eijck, J.R. Heringa, N.J. Steinke, F. Mulder, B. Dam, A.A. van Well The Journal of Physical Chemistry C 120 (19), 10185-10191
Contact information
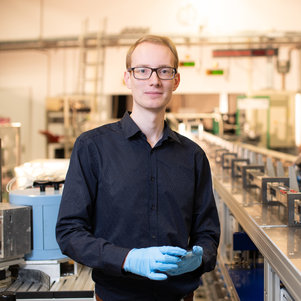
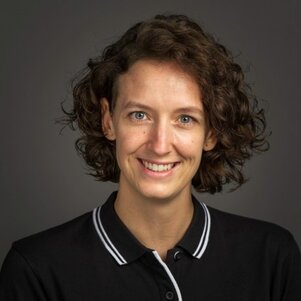